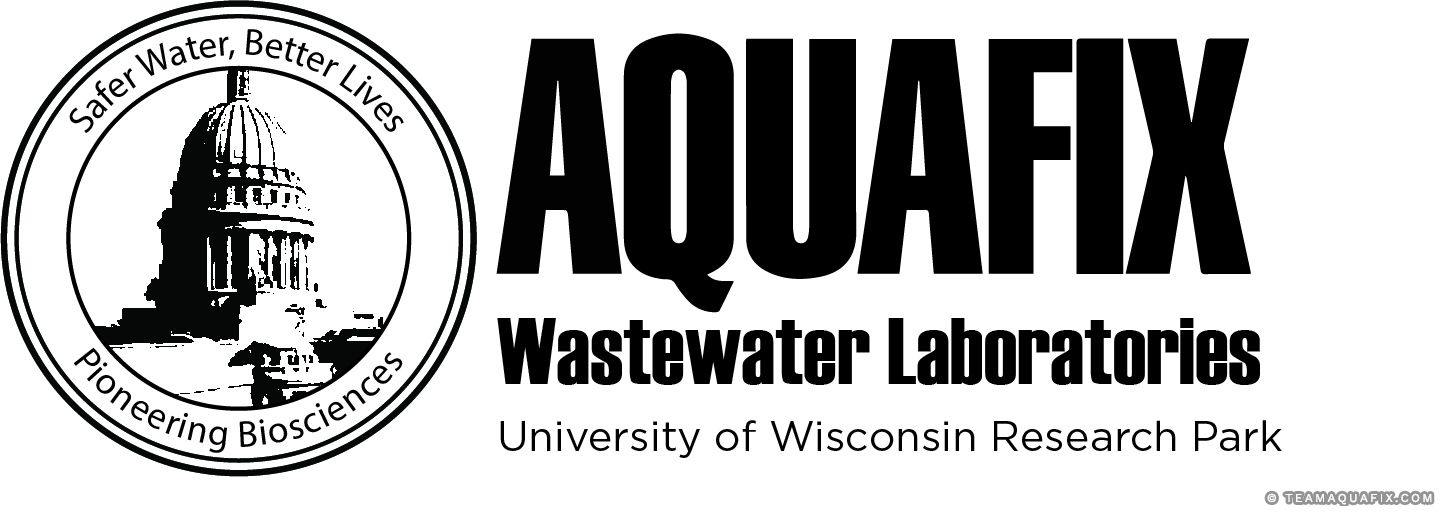
Nitrification in Wastewater Study
by Dan McKeaton, Chemist, Aquafix Inc
Summary
Nitrifying bacteria make up 4-6% of the population in an activated sludge process and have limited diversity compared to heterotrophic bacteria in wastewater. This makes them more susceptible to toxicity.
Nitrifiers have slow growth rates which means their population takes a long time to adapt to changes in environmental conditions.
This paper incorporates our lab and field data on VitaStim Dynamic Duo as well as suggested operating and environmental conditions for optimal nitrifier growth.
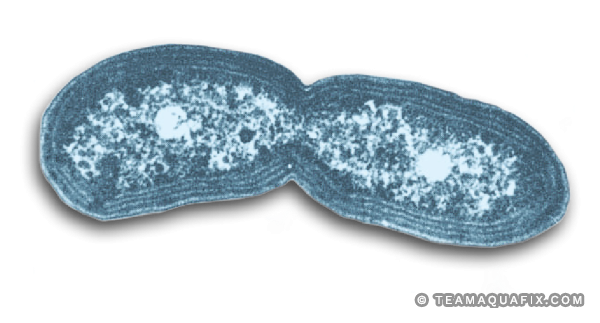
Abstract
Chemoautotrophic organisms, like nitrifying bacteria, produce energy through the oxidation of inorganic compounds such as ammonia and nitrite. This process tends to be significantly less efficient for energy production compared to heterotrophic energy production methods. Due to this fact, heterotrophic bacteria tend to dominate all nutrient removal until levels of BOD in a wastewater system become very low, and levels of dissolved oxygen increase. In addition, nitrifying bacteria are always present in lower concentrations and have lower diversity than heterotrophic bacteria.
Nitrifying bacteria are typically thought to make up 0.39–9% of bacterial populations in activated sludge (Yao & Peng, 2017). Most nitrifying activated sludge systems have 4–6% of the bacterial population made up by nitrifying bacteria (Yao & Peng, 2017). This lower population, growth rate, and diversity makes it much more likely that a healthy wastewater plant will have upsets that lead to poor ammonia removal rather than poor BOD removal. Nitrifier doubling rates are typically between 22–48 hours (depending on conditions), while heterotrophic doubling rates are typically between 20–30 minutes (Leech, 2019). Increasing the population of nitrifying bacteria through supplemental products such as VitaStim Nitrifiers is the fastest way to enhance nitrification in wastewater plants.
Results
Due to the fragility of nitrifying bacteria compared to wastewater heterotrophic bacteria, Aquafix Inc. has frequently worked with operators who have struggled to maintain nitrification in their wastewater systems and have developed our own nitrifying bacterial cultures, VitaStim Nitrifiers. This paper is intended to provide background information on nitrifying bacteria, ideal operational parameters for nitrifier growth, and lab- and field-testing results of our VitaStim Nitrifiers.
Nitrification is one of the two primary mechanisms for ammonia removal in aerobic wastewater systems. Nitrification is a two-step process performed by two categories of bacteria, ammonia oxidizers and nitrite oxidizers. Ammonia oxidizing bacteria (AOB) convert ammonia to nitrite through the ammonia oxidation pathway. This is followed by the conversion of nitrite to nitrate by nitrite oxidizing bacteria (NOB).
Ammonia oxidation and nitrite oxidation are thought to take place using several different enzymes. Ammonia oxidation begins by the enzyme ammonia monooxygenase (AMO) catalyzing a reaction of ammonia to NH2OH, which is then transported outside the cell where NH2OH is converted to nitrite using the enzyme hydroxylamine oxidoreductase (HAO) (Costa, 2006). Nitrite is then converted to nitrate by a reaction catalyzed by the enzyme nitrite oxidoreductase. These reactions provide the energy needed for nitrifiers to grow and reproduce.
Nitrifying bacteria are becoming increasingly better characterized, but more species are often discovered that are capable of nitrification. Traditionally Nitrosomonas (AOB) and Nitrobacter (NOB) have been considered the primary nitrifying bacteria present in wastewater systems, but there is increasing evidence that this is not necessarily the case. Different nitrifying bacteria tend to predominate to different conditions. This means different wastewater plants tend to have vastly different populations of nitrifying bacteria depending on the type of plant, operating conditions, and composition of wastewater. Some commonly studied genus of nitrifiers include ammonia oxidizing bacteria Nitrosococcus , Nitrosospira , Nitrosolobus , and Nitrosovibrio . Some commonly studied genus of nitrite oxidizing bacteria include Nitrospira , Nitrospina , and Nitrococcus .
Lab Testing of Aquafix Dynamic Duo Nitrifiers
Aquafix conducts ongoing testing and case studies to confirm the effectiveness of our nitrifier products. It is important to note, lab results and field results vary widely depending on the reason for loss of nitrification, the type of system present, and environmental conditions.
Our ammonia uptake testing involves adding a dose of our VitaStim Nitrifiers to a nitrifier growth media. Testing is run with limited light to prevent photoinhibition of nitrifiers on an orbital shaker plate set to 90 RPM in 500 mL Erlenmeyer
flasks containing 100 mL of our nitrifier media. Ammonia, nitrite, and nitrate are measured daily. pH is maintained at approximately 7.5 with the use of phenol red as a pH indicator in our setup and through the manual addition of sodium carbonate and hydrochloric acid as titrants. Testing, in this case, was run on nitrifiers that were stored in the refrigerator for approximately 6 months after growth in order to determine if nitrifiers remain active after this period. Nitrifier reactors were run in triplicate, while control reactors (no nitrifiers) were run in replicate.
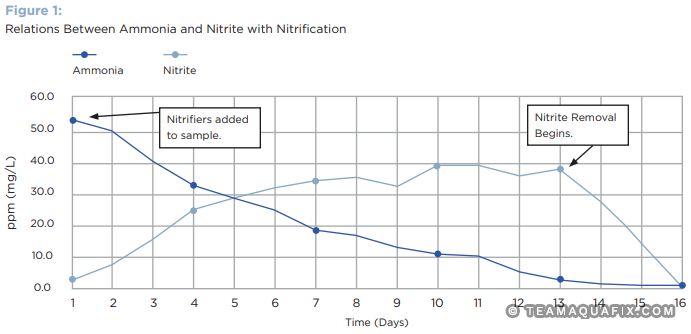
Our ammonia uptake testing (Figure 1) illustrates how growth of nitrifiers typically occurs in a controlled culture. Ammonia is converted to nitrite, then nitrate. As this test uses a tap water-based media without carbon and heterotrophic growth, pH variation tends to lead to slow growth of nitrifiers, and nitrite oxidizers. This testing confirmed that after 6 months in refrigeration, nitrifiers were still capable of removing ammonia in a solution. It also confirms the presence of nitrifying bacteria (AOB and NOB) in our nitrifier cultures based on the accumulation of nitrate.
Testing of Aquafix VitaStim Nitrifiers: Recovery from Toxicity
This testing was designed to assess if VitaStim nitrifiers were effective at reducing ammonia levels in our wastewater lab reactors after a toxic event that leads to loss of nitrification. Testing was performed in 500 mL Erlenmeyer flasks on an orbital shaker plate set to 160 RPM at room temperature (22°C). The mixed liquor and aeration influent used in testing was collected from a local dairy facility. Aeration influent came from two sources, a low strength waste discharge, and effluent from the anaerobic section of the system that treats the facility’s high strength waste.
The influent was autoclaved to prevent growth of bacteria throughout testing in the influent samples. High strength effluent and low strength influent were blended with dechlorinated tap water to produce a waste stream containing 450 ppm COD and 40 ppm ammonia. Reactors were initially prepared by diluting the MLSS to 2000 ppm with dechlorinated tap water, decanting 50% of the total reactor volume, and refilling 50% of volume with our influent blend. Flasks were fed this way daily for the duration of the testing. The pH in this test was measured and adjusted daily to 7.3 (+/- 0.1).
Reactors were run for 2 days, then a toxin, quaternary amine, was fed at 10 ppm on day 3 of the testing duration. VitaStim Nitrifiers were added to half of the reactors 2 days (day 5) later in a one-time dose of 20 ppm, along with 1 ppm of VitaStim Ammonia Assimilator. Both the control and nitrifier reactors were run in triplicate and averaged. Testing was run until full ammonia removal was observed in both the controls and the nitrifier reactors.
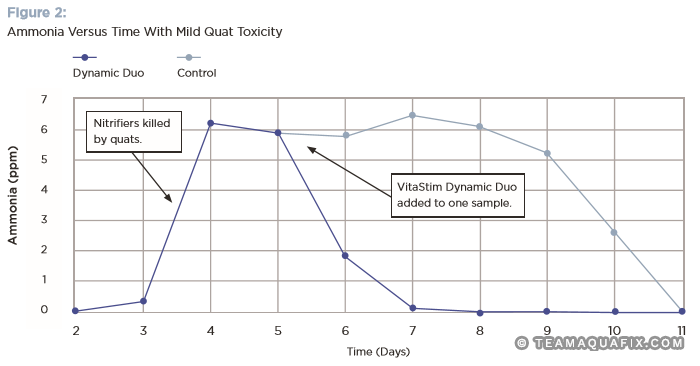
Figure 2 illustrates that the addition of nitrifiers was able to accelerate recovery of ammonia removal. In this case, Dynamic Duo was added on day 5 and complete ammonia removal was achieved in about one third of the time it took for the controls to fully recover. In more adverse conditions on the field (higher effluent ammonia, colder temperatures, variations in loading rates), full ammonia removal recovery would likely take more time to occur, both with added nitrifiers and with our controls, compared to this lab study, as will be illustrated in our included case studies.
Case Study 1: Pennsylvania Municipal System Diesel Fuel Spill
This municipal WWTP runs 318,000 GPD. Incoming BOD is typically around 220 ppm and incoming ammonia can be between 23–32 ppm. This plant contains two 334,000-gal SBRs (full) that decant down to 279,000 gals (about 16% volume) 4.5 times per day on average. In this system, an accidental spill of about seven 55-gal drums of diesel fuel in early November was discharged into the wastewater facility. The system cleaned up quickly but levels of ammonia in their plant effluent climbed up in November and continued to rise through December. Rebuilding the nitrifier population was exceptionally difficult as the temperature inside both SBRs was about 13°C. In the beginning of January, the effluent ammonia was about 6 ppm in both SBRs, which was also the facility’s effluent ammonia limit. At that time, the SBRs contained a MLSS of approximately 2,700 ppm in both reactors. This facility began feeding Dynamic Duo at a rate of 1 gal per SBR for the first week of January. By the end of the week, ammonia levels had returned to the typical 0.2–0.3 ppm usually seen in their wastewater effluent.
Case Study 2: Barge Cleaning Wastewater System in Texas
This industrial activated sludge system with three bioreactors treats 80,000–100,000 gal of barge cleaning wastewater per day. The bioreactors are arranged in sequence with two nitrification batch reactors. The first nitrification reactor typically has 250 ppm of incoming ammonia, which is reduced to around 25 ppm. The second typically reduces ammonia levels further down to about 1 ppm. The plant experienced a loss of nitrification, which was thought to be caused by toxicity. Ammonia in the plant effluent increased from 1 ppm to 25 ppm. Dynamic Duo was applied daily for a 10-day treatment at a rate of 3.5 ounces of nitrifiers daily to the first reactor. Ammonia levels recovered after 10 days to the 1 ppm typically observed in the plant effluent.
Case Study 3: Nitrite Lock in Hog Slaughter Facility in Iowa
Nitrite lock describes a build-up of nitrite caused by nitrite-oxidizing bacteria being unable to keep up with the levels of nitrite produced by their ammoniaoxidizing counterparts. This build-up can also then result in inhibition of ammonia removal. This can often occur in industries with high levels of incoming ammonia.
This case occurred at a hog slaughter facility with two anaerobic lagoons followed by an activated sludge process with a large 1.8 million gal aeration tank with a 2-day hydraulic retention time (900,000 GPD flow). Wastewater entering the aeration tank contains low levels of BOD (30–40 ppm typically), but high ammonia (200 ppm incoming ammonia). Ammonia in the aeration effluent is typically below 1 ppm, with nitrite levels below detection limits. In early October, this plant experienced heavier than typical loading, causing pH to drop to 6. In addition, an error left a wasting pump on for 24 hours, leading to significant loss of solids. Initially, ammonia spiked up to 5.86 ppm but returned to normal within a day without treatment. However, nitrite levels in the basin rose to around 45 ppm. The facility started by adding 2 gal of Dynamic Duo, and after 1 day their nitrite levels decreased to 7.7 ppm. A temperature shift the next night led to nitrite levels in the system increasing back to 32 ppm. As treatment continued, nitrite levels fell to 7.5 ppm after 1 more day of treatment and recovered to 0.199 ppm nitrite in effluent after 3 more days.
Important Operating Conditions for Nitrifiers
Nitrification becomes the primary form of ammonia removal after levels of BOD have been exhausted. Ammonia is often released in the decomposition of urea but will also appear in lower quantities due to the degradation of proteins and other nitrogen-containing molecules. Organic forms of nitrogen, such as amino acids and proteins, tend to be favored forms of nitrogen for heterotrophic bacteria. This means in wastewater treatment systems some excess ammonia is often present that must be oxidized by nitrifiers to achieve effluent limits for ammonia. In cases of lost nitrification, this extra ammonia is what is observed in waste discharge.
In wastewater treatment systems, nitrifiers tend to be poorer floc formers compared to heterotrophic organisms due to lower extracellular polymeric substance (EPS) production. This means proper floc formation by heterotrophic bacteria leads to more stable nitrifier populations as EPS generated by heterotrophs helps to anchor nitrifiers in a wastewater system.
As previously mentioned, different types of nitrifying bacteria can tolerate and thrive despite significant variations in environmental and operational conditions. Several operational considerations can improve nitrifier diversity and maintain a larger population of nitrifiers to minimize the risk of losing nitrification.
1. Retention Times
Typically, an HRT of greater than 10 hours is recommended for nitrification and a greater than 8 days MCRT is considered ideal for maintaining an adequate nitrifier population. This often takes more time in systems with adverse conditions, which leads operators to increase their MCRT during adverse conditions such as low temperature. If the HRT is too short, nitrifiers will not have enough time to perform ammonia removal after the heterotrophs have finished BOD removal. If MCRT is too short, then too many nitrifiers will be removed from the system to allow them to maintain a population. Keep in mind, if MCRT is too high, poor flocculation and filamentous growth will occur. It is often recommended to maintain a MLSS above 2000 to adequately protect nitrifiers from adverse conditions and maintain a nitrifier population that can withstand stressors such as temperature changes (Gererdi, 2002).
2. Temperature
Nitrifiers prefer a temperature range between 15–30°C. Nitrifiers have trouble growing fast enough to maintain a population below 15°C, and sometimes have problems with low dissolved oxygen levels above 30°C as oxygen solubility decreases in higher temperatures. Nitrifiers have been recorded functioning effectively outside this temperature range as the bacterial population is often able to acclimate to varied temperatures. Rapid temperature changes also have significant adverse effects on nitrification since nitrifiers are unable to adjust quickly due to their slow growth rates. Nitrifiers have been observed functioning over 35°C (as high as 52°C) in a recent study (Knight, 2019), which had been thought to be near impossible.
3. Dissolved Oxygen
Nitrifiers in wastewater plants function optimally when dissolved oxygen is present between 2–3 ppm. The main reason for this is that DO in wastewater systems tends to be maintained around this point for effective aerobic activity. Some species of nitrifier have been known to thrive well below 1 ppm of DO, and many nitrifiers present in natural aquatic environments prefer higher levels of DO as their environments typically have very low levels of BOD. Due to these factors, if reseeding a nitrifying population, it is important to use nitrifiers that are acclimated to the DO levels of a typical wastewater treatment plant. Nitrifiers used for other applications, such as aquariums, may not be as effective in wastewater systems due to their preference for higher dissolved oxygen levels.
4. Alkalinity
Alkalinity is one of the most important environmental factors for nitrification for two major reasons. The first is nitrifiers use dissolved carbon dioxide, carbonate, and bicarbonate as their carbon source for autotrophic production of glucose, and (CO2, HCO3-, CO3-2) are major contributors to a system’s alkalinity. The second reason is nitrifiers produce nitric acid during ammonia oxidation and if alkalinity is low, pH fluctuations due to acid production can lead to poor nitrifier growth. This means in cases of low alkalinity, it is much more beneficial to supplement alkalinity with carbonate or bicarbonate, rather than other commonly added basic compounds such as sodium hydroxide or magnesium hydroxide. Typically, an alkalinity around 100 ppm is optimal for nitrifier function but there is no harm in being lower or higher assuming the system is maintaining a stable pH and nitrification is functioning well enough to handle the rate of ammonia loading in a system. 8.64 mg/L bicarbonate (HCO3) is considered adequate to remove 1 ppm of ammonia in wastewater systems based on a model of nitrification from 1976 (USEPA, 2002).
5. pH
Nitrifiers prefer a neutral to slightly alkaline waste stream (pH 7–8). At lower pH values (6–5.5), nitrifiers grow and operate more slowly, and at higher pH values (9–9.5) nitrifiers will begin to die in a system, making it difficult to maintain an effective population. If alkalinity is properly maintained, it is unlikely that pH values will be outside this range in most wastewater systems.
Conclusion
Nitrifying bacteria are an essential part in achieving near complete ammonia removal in activated sludge systems. In many cases, wastewater systems with effective nitrification can achieve ammonia and nitrite levels below detection limits. Nitrifiers require specific operating conditions to function effectively and are more sensitive to toxicity and other adverse conditions than most wastewater heterotrophs. If optimal conditions are present in a wastewater plant, nitrifier populations will be more robust and less susceptible to upsets. If adverse conditions do occur, and nitrification is lost, VitaStim Nitrifiers and VitaStim Ammonia Assimilators help to quickly recover a nitrifier population to avoid effluent limit violations.
Thank you for sharing this fascinating blog post! The lab testing of Aquafix Dynamic Duo Nitrifiers is intriguing. It’s essential to explore innovative solutions for nitrification in wastewater treatment. Your insights are valuable, and I appreciate the informative update. Keep up the great work!